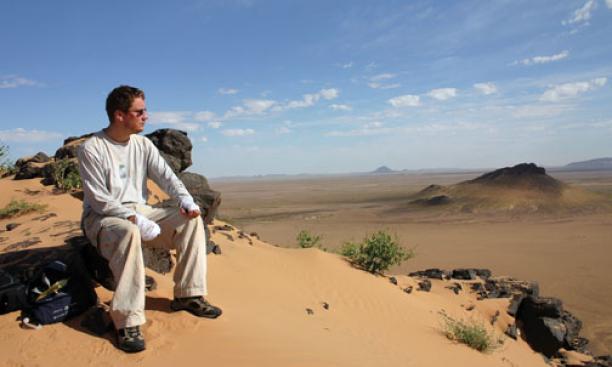
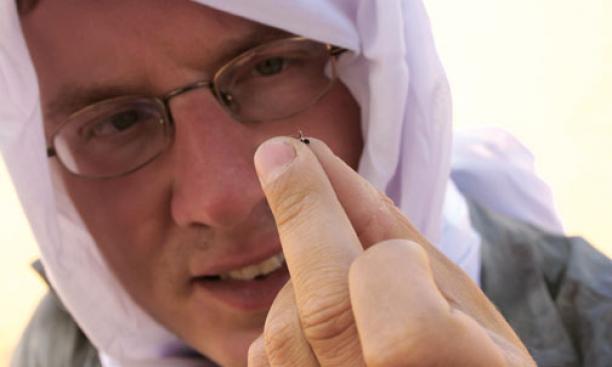
Say what you will about the ineptitude of a herd mentality. For Iain Couzin, herds — and flocks, swarms, and all varieties of crowds — are worthy of respect.
Since 2007 an assistant professor of ecology and evolutionary biology at Princeton, Couzin (pronounced “cousin”) has made major contributions to understanding collective animal behavior. Why do billions of locusts suddenly begin to move? How do ants keep on marching — on densely packed trails and carrying heavy loads — without congestion or the slightest hint of road rage? How do flocks of birds select their navigators? And what about those strange creatures — humans — as they make decisions as citizens, drivers, or in a variety of other situations?
Many of the big issues in biology today concern collective systems — from cells, to animal collectives, to entire ecosystems. Couzin’s work has implications for fields ranging from ecology to cancer research to brain function and human behavior. But for him, it all began with animals. As a kid growing up in Scotland, he was fascinated not by dogs, cats, or traditional pets, but by ants and other social insects, which had the added advantage of not provoking his brothers’ allergies. How they functioned together as a small society always intrigued him, he says. Then, after his first biology lecture in college, at the University of St. Andrews in Scotland, he wrote to his parents and told them he knew he’d found his passion. “The lecturer made it so clear that so little was known, and that there was so much to be discovered,” says Couzin.
“I always thought that flocks of birds and schools of fish were so interesting,” the scientist says. “I just assumed there was a whole discipline that was dedicated to [those collective movements].” There wasn’t, at least nothing that rigorously tested hypotheses about how and why these animals were able to move in harmony, often at lightning-fast speeds (think of a school of fish cleaving and reforming in response to a predator).
Flocks, schools, and swarms may form similar-looking patterns, but the driving forces behind them are often different; it’s important to understand the inner workings of animals and the evolutionary forces that might have produced their behavior in order to fully understand it. Yet the study of an individual animal — while essential — isn’t enough to understand its role in the greater group. “You can dissect a locust and learn nothing about why they swarm,” he says.
Couzin began with biology, but it soon became clear, he says, that the traditional tools of his field were insufficient to answer the questions about collective behavior in which he was most interested. “Verbal arguments and even classical experimentation wasn’t going to be specific enough — we needed a framework for discussion,” he says. For that, he had to program computers to capture individual animals’ motions, run simulations that might explain what rules govern their behavior, make predictions about those rules and their results, and test those predictions in experiments.
Because of his biology course load, Couzin hadn’t had much opportunity to delve into math and computer science in his undergrad years, so he sought it out in his graduate work and, he says, “got hooked.” Not that computer programming was easy (he didn’t even have e-mail when he started working on his Ph.D. at the University of Bath), but it allowed him to reach levels of analysis that weren’t possible before.
For his doctoral research, he studied the movement of army ants in Panama. “My original observation was that they formed lanes,” says his adviser, Nigel Franks, now a professor of animal behavior and ecology at the University of Bristol in England. Two outer lanes were filled with ants moving at a rapid clip to reach the food source, following a chemical trail laid out by scouts, and a single inner lane was taken up by slower-moving ants returning home with prey. “What Iain did was to think hard about what rules they might be using,” says Franks. By filming the ants, and then doggedly advancing the video frame by frame to track their movements, Couzin created a computer model to simulate what would happen under different scenarios — allowing him to see much more than by observing a colony with hundreds of ants doing things at the same time. “He was able to show that the rules were beautifully tuned to create the most smooth” traffic flow, says Franks.
Couzin and his former postdoc adviser, Simon Levin, George M. Moffett Professor of Biology at Princeton, became interested in collective activities in general. “We wondered how consensus is reached in groups in which there are heterogeneous opinions and in which most individuals don’t have any opinions at all,” says Levin. If you’re thinking that sounds like the American electorate, you’re not far off, but the paper that ultimately was published in (and made the cover of) Nature in 2005 developed a model based on animal groups on the move. How could flocking birds or schooling fish, for example, travel in unison without recognized leaders? The researchers — Franks and Jens Krause of the University of Leeds, along with the Princeton scientists — used computer simulations to show how coordination arose. First, the instinct to stay in a group — straying could be deadly — causes animals to stick together, but not so closely that they’d collide. Second, some animals in the group have information about where they need to go — to find food, for example. The scientists showed that only a few individuals need to have preferences as to which way to go. (Think of the small proportion of bees in a hive who do the “waggle dance” to inform other members of the location of food.) In fact, the “larger the group, the smaller the proportion of informed individuals needed to guide the group with a given accuracy,” they wrote.
The uninformed masses, however, are very important. “They provide a lot of momentum,” explains Levin. “As they shift from position A to position B, the whole population shifts.” Of course, a single model can’t explain both how bees swarm and politicians swing 20 points in the polls over a month. (For one, bees are so genetically similar to each other that they don’t pursue their own selfish interests. The American public is another matter.) But the researchers wanted to pare down such collective activity to the simplest possible model. And their findings had implications for how other researchers looked at their own work.
“Many biologists are fascinated by complexity,” says Martin Wikelski, a former Princeton professor who now directs the program in migration and immuno-ecology at the Max Planck Institute for Ornithology in Radolfzell, Germany. “Iain is fascinated by the simplicity, which explains and informs the complexity.” Based on Couzin’s ideas, Wikelski says, “we have now started exploring whether global animal migrations may largely be explained by very simple rules of thumb.” Birds flying south in the autumn, for example, used to be thought to possess some kind of incredible navigation skill, such as following the stars. “Now, we can imagine alternative explanations; perhaps all a bird needs to know is that at a certain time of year I have to listen to what everyone else is doing and to follow the flow southward. Orientation and navigation seem much easier when you understand that you can get information from other organisms [around you],” Wikelski says.
Couzin also has worked to understand the swarming behavior of locusts, an obvious — and destructive — example of collective animal behavior. Being able to predict such swarms would be a boon for human populations in their path. But until recently, no one knew exactly what made locusts hit the road. Tying into parallel work they did on other mass-marching insects (Mormon crickets in the western United States), Couzin, along with collaborators Stephen Simpson and Greg Sword, both now at the University of Sydney in Australia, and Princeton graduate student Sepideh Bazazi, explored what made the locusts tick. Their work spanned observation in the field (Couzin says one multiweek trip to Mauritania included relying for sustenance on a sort of jerky made from dried camel entrails), a “locust marching arena” in the lab for direct experiments, and computer simulations. Their attention-getting conclusion, published in Current Biology in 2008, was cannibalism: The locusts were moving forward to avoid being eaten from behind by a not-so-friendly neighbor-locust hungry to replace depleted protein and salt stores. All it took was a tickle from behind, the scientists found, to motivate the insects to aggregate and march forward.
Couzin’s essential contribution to the project was the image analysis and computer models, which succeeded so well because of his training as a biologist. “It’s very easy to derive simulation models to the nth degree,” Simpson says. “Relating those to real systems is the challenge; you have to understand how real organisms work and interact together.”
One recurring theme in Couzin’s research is working with colleagues across disciplinary lines, which he says “just naturally happens” at Princeton. “We come from completely different universes,” says Bernard Chazelle, a computer science professor. “I’m a theoretical computer scientist, working on algorithms and complexity theory. He’s a biologist. But the kinds of questions he asks match to a surprising extent many of the most interesting questions in my field.” Chazelle recalls going to see a lecture Couzin gave to the computer science department. “I was listening to this guy talk about locusts and birds and fish, and what I was hearing was a story about algorithms — one after the other! It was really inspirational,” he says. The professors’ research agendas haven’t given them enough common ground to collaborate formally, but Chazelle believes there’s potential: After all, the natural world is full of feats that humans cannot duplicate despite their knowledge of math and physics — engineers cannot create a plane that behaves like a bird, for example. He’s hoping algorithms can help divine some of those rules and give us predictive tools.
Couzin is working with Naomi Ehrich Leonard ’85, the Edwin S. Wilsey Professor in the Department of Mechanical and Aerospace Engineering. She’s interested in developing mathematical models and tools to help explain how animals perform group activities like foraging for food and to bring new insights to the design and analysis of collective behavior in robot groups. This may help Leonard program the underwater robotic vehicles she works with to forage most efficiently for information in the sea. “Iain talks about individuals using a minimalist approach” to behaviors like seeking food, she says. “That’s very appropriate for robotics.” If, for example, effective foraging only requires a few informed individuals, that means a group of robots could include some without expensive sensory systems. “We are both ultimately interested in understanding how to connect these relatively simple decisions that individuals make in response to what they sense in their environment to the complex behavior at the level of a group,” Leonard says.
She and Couzin also have developed a test bed, using imaging software he developed, that integrates real fish with robotic fish, programming the robots to act in a certain way and seeing how the actual fish respond. That, she says, allows them to potentially explore the dynamics of a school using the researchers’ own “infiltrators.” “We’re not just using biology to understand the robots,” Couzin says, “but we’re also using the robots to understand biology.”
There are plenty of other research opportunities, too: Last year Couzin co-authored a paper hypothesizing that cancer cells migrating during metastasis — when the cells leave the primary tumor and journey elsewhere in the body — may have some parallels to animal swarms, in the way the cells collectively can sense the environment and make decisions in response to it. It’s very early theoretical work, but it does point to a new angle for cancer research: trying to knock out the mechanisms behind such collective migration. In another paper published last year, he proposed that the same framework used to “decipher the relationship between individuals and group-level properties” may help us understand how brain cells work together to produce a single mental action.
Of course, modeling different organisms requires different algorithms, as Couzin explained to an interviewer with the nonprofit Edge Foundation (the interview, at edge.org, is titled: “Ants Have Algorithms”). Most of the worker ants doing the heavy lifting are females who are very closely related to each other, and so have much more genetic “interest” in working together than fending for themselves alone or for their direct offspring. So, as Couzin points out, “of course they function as colonies.” He went on: “When we look at the optimization of an ant trail, we can look at what optimizes the benefit for all of the individuals. We also model human crowds ... and we use a different algorithm entirely — we have a game-theoretic algorithm where individuals may minimize their own travel times, but may do so at the expense of others because they don’t necessarily care whether they cause congestion for other individuals.”
Katherine Hobson ’94 is a journalist who writes about medicine and health.