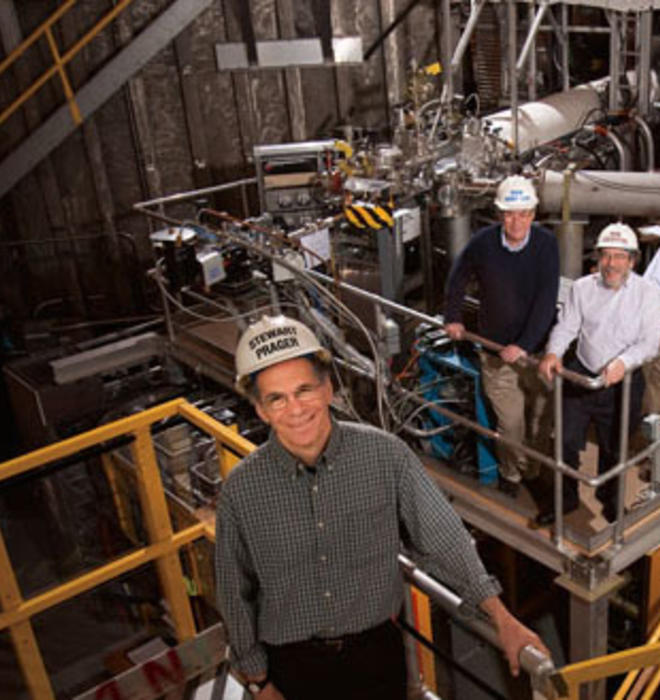
Firing up fusion
In a resurgence of worldwide fusion research, the Princeton Plasma Physics Lab tries to carve out a starring role
The quiet room has the airless feel of a mausoleum. Hutch Neilson, the former project manager for the National Compact Stellarator Experiment at the Princeton Plasma Physics Laboratory (PPPL), is standing in a cavernous, concrete-lined bunker at the lab. Stacked around him are the plywood boxes and plastic-wrapped remnants of what was, for him, his baby — strange-looking, perhaps even mutant, but no less dear because of it. Neilson peels the plastic from one of the 6,000-pound steel forms and admires its craftsmanship: the bizarrely sculpted surface, shaped to within 3/8ths of an inch to hold powerful magnetic coils precisely in place. The room contains $90 million of mothballed work. But the loss Neilson feels is not mainly about money. The work represents the dedication of scientists who see a future powered not by wind or water or even the rays of the sun. They see a future powered by a sun-on-Earth: fusion, the nuclear fire of stars. The stellarator — known as the NCSX — was supposed to stoke that fire within its peculiarly shaped core. Neilson touches a steel flange, checkmarked by a quality-control inspector’s felt-tip pen, and considers the decade of his life spent on the project. “One has to move on,” he says.
In May 2008, the U.S. Department of Energy canceled the stellarator, PPPL’s crown jewel, even as it had passed the halfway point in its construction. The culprit was its ballooning budget, which had swelled from $102 million to $170 million. Times were tough for most fusion scientists: The world’s great hope for fusion research, an international reactor in France called ITER — the largest and most advanced in the world — was far over its €10 billion budget, years behind schedule, and facing a major design change (see related article, on page 27). In the United States, the Department of Energy’s fiscal-year 2008 budget for fusion had been slashed dramatically, ending U.S. contributions to ITER. Meanwhile, Princeton, which manages PPPL for the Energy Department, was in the process of competing for a five-year contract renewal. Longtime director Robert Goldston *77 stepped down so that a new director could be a part of the contract competition. “It couldn’t have been a worse time to compete for a new contract,” says Princeton’s dean for research, A.J. Stewart Smith *66, who oversees the lab. “It was a perfect storm.”
Though the Energy Department is being overhauled to reflect the renewable-energy priorities of President Barack Obama’s administration, it is unclear where fusion fits within the overall vision. There are some hopeful signs: Funding for ITER was restored in fiscal-year 2009, and a $3.5-billion fusion machine that uses a different approach — powerful lasers — was dedicated in May at the Lawrence Livermore National Laboratory in California (the technology has implications for nuclear weapons, as well as energy). Still, critics have argued that fusion always seems to be a decade or two away, but never any closer to reality. Stewart Prager, who began work as PPPL’s new director Jan. 21 — less than a week after the Energy Department renewed its contract with Princeton — will have to counter this thought if he is to put PPPL back on the map as a way station en route to viable fusion. The longtime director of a fusion center at the University of Wisconsin in Madison, Prager has seen fusion budgets rise and fall — usually in sync with the price of oil. Expensive oil tends to remind bureaucrats that the world eventually will need a limitless energy source. “There is a fundamental need for an abundant, clean, and safe energy supply available to all nations,” says Prager, using a well-rehearsed line, but one that he utters no less earnestly. Fusion may not make economic sense now, and it may not yet be ready in 30 years. But eventually, he says, it has to happen. The goal of fusion power, he says, “transcends economics.”
Since the dawn of the nuclear age, fusion has been a sort of holy grail. First came the discovery of fission, in which heavy radioactive atoms like uranium, split by neutrons, give off heat. While nuclear reactors have tamed fission into a viable energy source, the by-products are radioactive, and persistently so. Also, reactors can breed and enrich their own fission fuel — a good thing for the reactor operator, but bad for those who want to clamp down on the proliferation of bomb-grade uranium and plutonium produced in the guise of a civilian program.
Fusion, on the other hand, is pretty much as Prager describes it: abundant (the basic fuel is heavy isotopes of hydrogen, easily obtained from seawater), clean (the only radioactive ingredient is tritium, a short-lived heavy hydrogen that’s easily cleaned up), and safe (there is no chance of runaway reactions, nor can the technology be siphoned off into the production of a bomb). Plus, like solar, wind, and hydropower, it doesn’t emit carbon.
But those other renewable energy sources will require the development of a large number of available sunny desert spaces, windy plains, and steep river gorges. The basic fuel for fusion, however, is essentially limitless. In just a tablespoon of seawater there is the fusion-energy equivalent of a gallon of gasoline.
The only problem with fusion is that it is an extraordinarily tough thing to do. It requires sunlike temperatures and pressures to convince hydrogen atoms to do something awkward: fuse into helium. Stars get around this problem by being big: They are so heavy that their own gravity does all the necessary squeezing. On Earth, it’s more difficult. Fusion occurs when the atoms are in a hot plasma — a state of matter where ionized gases behave somewhat like a liquid. One can heat the plasma so the hydrogen atoms strike each other fiercely enough to fuse, but that reaction releases extra energy, agitating the atoms all the more. They move faster, try to expand, and therefore are more reluctant to fuse. The atoms need to be confined — but few materials would last long touching a 100 million-degree-Celsius plasma.
However, since plasma is charged, it can be moved and controlled at a distance by magnetic fields. Because of this, many scientists, including those at PPPL, think the best way to contain plasma is to create hollow structures ringed by magnetic coils, such as the doughnut-shaped tokamak machine. The tokamak is one particular shape and approach, but there are variations. There are stellarators, invented by Lyman Spitzer Jr. *38 (PPPL’s founder and first director) — supposedly, while he was daydreaming on a ski lift. A stellarator is like a twisted tokamak, and the NCSX is one particular incarnation (millions of slightly different twisted stellarator shapes are possible). Stellarators add a degree of complexity to the magnetic fields in return for eliminating the need for the plasma to be moving within a tokamak. A stellarator plasma is thought to be less susceptible to turbulent disruptions and more conducive to steady-state operations. But a third approach ultimately could win out: This one is similar to a tokamak, but fatter, with the central hole of the doughnut so small that the overall structure is better thought of as a sphere. Used in facilities such as PPPL’s National Spherical Torus Experiment (NSTX), spheres can maintain higher-pressure plasmas with lower magnetic fields. This means fusion can occur in smaller containers using less electrical power.
So far, tokamaks seem to be the most likely way to go, and using them, fusion scientists have made great strides, says PPPL deputy director Richard Hawryluk. PPPL’s Tokamak Fusion Test Reactor (TFTR), which shut down in 1997, produced tens of megawatts of power for seconds on end — millions of times more energy than that created by early-era machines — and neared the “break-even” point, where output energy from fusion would surpass the input energy needed to heat up the plasma. By another measure — a combination of the heat, density, and duration of a plasma — fusion reactors have improved faster than Moore’s Law (which said that the number of transistors that can be placed on a computer chip would double about every two years). “The long view is that we’ve made unbelievable progress,” says Hawryluk. ITER should be the machine that achieves a self-sustaining chain reaction called “burn” or “ignition,” where no heat has to be added in order to achieve fusion. A demonstration power plant could come next, with the ability to burn plasma continuously, rather than in bursts. That, Hawryluk says, could happen by 2040.
Yet the perception has been that progress toward fusion has been slow — perhaps because the goal is so big. Prager says the situation wasn’t helped by quasi-research into “cold fusion” and “bubble fusion” — both of which made claims that fusion eventually might be achieved on a tabletop. Those “fiascos,” Prager says, branded all of fusion. Even now, many in the public confuse cold fusion with hot. “It’s almost like confusing astrology and astronomy,” he says. But he acknowledges that fusion scientists need to be better at explaining their progress, calling for patience, and noting that good things can come from the research along the way — such as the way the semiconductor industry has learned to use plasma to finely etch its microchips. Fusion scientists would be wise to imitate the cancer-research community, which, Prager says, manages to explain the advances and side benefits of its work well enough for the public to understand. “[People] don’t simply ask, ‘When are you going to cure cancer?’”
Prager also is calling for patience among his own staff. As one of the Energy Department’s 10 scientific national laboratories — and the only one completely devoted to fusion — PPPL’s place is secure; its staff of 425 and its $81 million budget haven’t changed in half a decade. Some in the lab want Prager to lobby for a major project to replace the void left by the NCSX cancellation. But instead of putting all the eggs in a single basket, Prager is preaching diversity: He wants PPPL to pursue projects of different sizes — small, medium, and perhaps large — and to concentrate on developing niche expertise. The brainpower, rather than the machine power, is what is important, he believes. As longtime PPPL scientist Ned Sauthoff, who now leads U.S. ITER efforts at Oak Ridge National Laboratory in Tennessee, says, “Research institutions should not be owned by their iron.”
PPPL already is pursuing a handful of small-scale experiments that cost on the order of $10 million. One with particular promise is the Lithium Tokamak Experiment (LTX), a spherical torus small enough to fit in a pickup truck. Almost accidentally, it was discovered that lithium — that age-old treatment for depression — also seemed to have a way of soothing red-hot plasmas. “It was almost like throwing cold water into the plasma,” says LTX scientist Phil Efthimion. The lithium allowed for a thicker, more manageable plasma and longer burns. A previous version of LTX just used a tray of solid lithium at the bottom of the tokamak, but Efthimion is now working to run LTX with a thin liquid coating of lithium clinging to the entire tokamak wall — which could further enable lithium’s seemingly magical powers. “It could have a big impact,” he says.
Around the medium range — on the order of $100 million — are experiments such as NSTX, which was like the yin to the stellarator’s yang. When NCSX was canceled, the spherical experiment was given the green light for continued operations and today is in the running for a major upgrade, which would double the strength of its magnetic coils. For now, NSTX project director Masayuki Ono *78 says, the team is trying to make the most of 2009’s 16 weeks of approved operation. “It’s still relatively new. We’re gaining confidence every day.” Of course, many at PPPL still nourish the dream that NCSX will be resurrected — one reason why the lab mothballed the parts so carefully. While Germany and Japan are each building a stellarator, no other facility in the United States has embarked on a big one, and the particular issues explored by stellarators have not disappeared.
But PPPL lacks a large, billion-dollar project on the scale of the machine it had in the 1990s with TFTR. And, Prager notes, it’s unlikely to get anything like it in the near future. But that shouldn’t stop the lab from planning for one. Former director Goldston, who has remained on the staff to — among other things — lead PPPL’s ITER involvement, already has an idea. ITER is expected to produce 500 megawatts of power in bursts of fusion lasting six to seven minutes — a major step, but still short of the 2,500 megawatts that a demonstration fusion power plant would be expected to produce continuously. In ITER, hot particles from the plasma slam into a particular part of the tokamak called the diverter — and the diverter barely can handle it. Diverters are expected to wear out quickly. “The technology has to change,” says Goldston, who wants to build a small tokamak that mimics the high power of a demonstration reactor. PPPL then would use its expertise with lithium technology to experiment with the materials and technology needed to withstand those conditions.
Eric Hand ’97 is a writer for the journal Nature.
It has been a long road for ITER, the machine that could bring the fusion burn of the sun to a place not too far from the French Riviera. But if it is to succeed, there may be a detour along Route 1 to pick up a few parts made in New Jersey.
Originally conceived in 1985, ITER stands for International Thermonuclear Experimental Reactor. Since “thermo-nuclear” tends not to get the best public relations — the word seems to suggest a bomb, rather than a balm for mankind’s energy troubles — officials refer to ITER by its acronym alone, noting that ITER is Latin for “the way.”
But “the way” has been littered with obstacles. For many years, politicians couldn’t even decide on a place to put it, primarily because of squabbling between Japan and France, each of which wanted to host it. Finally, in 2006, seven parties — the United States, the European Union, Russia, China, India, Japan, and Korea — signed a treaty that officially formed ITER. Clearing at the selected French site, in Cardarche, has begun, and construction is expected to start next year, with experiments beginning by the end of the next decade. But the €10 billion price tag estimated in 2001 — about $13 billion — could end up nearly doubling in a revision due before the end of this year. And a new problem controlling the plasma is forcing designers to add another layer of magnets at a late stage.
ITER’s doughnut-shaped tokamak design calls for giant superconducting magnets to keep a handle on hydrogen plasma heated to temperatures of 100 million degrees Celsius. ITER — by far the biggest fusion reactor ever attempted —- would be the first to reach “ignition” and “burn,” the point at which heat created from fusion is sufficient to sustain the reaction.
With fusion budgets that have been smaller than those in other participating nations, the United States never really was in the running to host ITER. Still, PPPL was, for many years, the locus of this country’s ITER involvement. Then, at the beginning of 2006, two years before the stellarator was axed, the Department of Energy decided to move U.S. ITER headquarters from PPPL to Oak Ridge National Laboratory in Tennessee. PPPL lost U.S. ITER director Ned Sauthoff, a longtime employee who decamped for Tennessee. “I’m sure that Princeton would have liked to have kept its position as lead and host [for ITER],” says Sauthoff, who attributes the Energy Department’s decision to Oak Ridge’s project-management experience in completing a major neutron-beam facility. To stay influential on ITER, Sauthoff says, Princeton will have to carve out niches, specializing in smaller, but still crucial, activities.
A good example of the Princeton lab’s relevance lies in the latest issue ITER’s designers have encountered: ELMs, an innocuous-sounding acronym — but potentially devastating problem — that stands for Edge Localized Modes. ITER’s huge size helps it contain larger plasmas at higher temperatures, but it also means the plasma is more unruly. An ELM, a particular type of plasma turbulence, is like a puncture wound at the edge of what is supposed to be a smoothly confined plasma wall.
Wearing a hard hat, Masayuki Ono *78 walks into the control room at the Plasma Physics Lab for the spheroidal torus experiment (NSTX). He opens his laptop to show what an ELM is like. Ono plays a video of a few seconds of NSTX operations, captured by a camera capable of gathering 100,000 frames per second. The video frames are like global X-rays of the sphere. All is quiet and black until a burst of light suddenly appears in one corner and spreads, blanching the globe in white. “You see it brighten up?” asks Ono. “That was an ELM.” In a fraction of a second, the ELM deposits about 20 kilojoules of energy — 10 percent of the NSTX’s output — into the machine’s wall. That’s less than the heat energy in a teaspoon of gasoline, so the NSTX can handle it, says Ono. But ITER may spew ELMs a thousand times more destructive, tossing out the equivalent of a few hand grenades every second. “You can imagine what that would do to the walls,” he says.
6 Responses
J. Charles Nickerson ’65
8 Years AgoJohn Sethian ’72
8 Years AgoEric Hand ’97’s “Firing up fusion” hits home; I have worked on fusion since my senior thesis at Forrestal long ago. Technical and scientific challenges are indeed a factor in the long time to develop fusion. But another is the need for a policy that develops fusion energy as an integrated system and fosters competition among fusion approaches.
Realizing a fusion power plant requires connecting pure science, applied science, and engineering together as an integrated system. Understanding fusion plasmas, as done at PPPL, is crucial. But the current policy virtually ignores the other needed technologies. These include neutron-resistant materials and efficiently heating and containing these plasmas. Ultimately it is these technologies that will determine if fusion leads to an attractive power plant, and they need to be developed concurrently.
Stifling competition hampers innovation, slows progress, and increases risk. If fusion has such potential, why pursue just one approach?
One alternative is “inertial confinement,” in which lasers are used to initiate the fusion reactions. The Department of Energy vigorously pursues this to study the physics of nuclear weapons. Energy development is carried out only because of congressional direction. The Naval Research Laboratory (NRL) is leading that program. The key science and technologies are developed in concert by a team from 25 U.S. institutions. Of course, PPPL scientists and engineers are part of that team. Enough progress has been made that it may be possible to demonstrate net fusion power much sooner than originally thought. But an investment in this approach requires a change in policy.
Many talented scientists are dedicated to harnessing fusion energy. We can make it happen. But we need a policy that enables us to reach that goal. I am cautiously optimistic that the new administration may be headed in that direction.
Stuart G. Hibben ’48
8 Years AgoThe news that the Princeton Plasma Physics Lab (PPPL) is back in full action again (cover story, June 10) is the best from Princeton in recent memory. Despite the lost “mothball” years, I am confident that it will again play a lead role in the development of fusion power.
The potential benefits of fusion power are incalculable. To cite just one, it may become the solution to the problem of world population, which is now growing at an unsustainable rate. This linkage was suggested a generation ago by the late futurist Herman Kahn at the Hudson Institute, who surmised that cheap commercial fusion power would become a practical reality in this century, available worldwide.
This should lead to a general rise in the standard of living, notably in Third-World countries. Kahn observed that, historically, higher standards of living have resulted in lower birthrates. Barring natural or man-made disaster, Kahn’s model thus shows world population peaking and then leveling off after fusion is in place. What a wonderful legacy that would be for the fusion pioneers!
We should all be pleased and proud that PPPL is once again fully engaged in this vital effort.
J. Charles Nickerson ’65
8 Years AgoHaving been a student of technical subjects at Princeton (and then Stanford) in the 1960s, I was conversant with, and at least mildly excited by, the promise of nuclear fusion. I am also bipolar, and was amused by the fact that lithium, which has helped me immensely, seems also to help, may I say, “bipolar” fusion plasmas (cover story, June 10). Apparently, lithium is not enough to solve all the plasma instabilities. Other psychoactive drugs might be tried with even greater success!
John Sethian ’72
8 Years AgoEric Hand ’97’s “Firing up fusion” (cover story, June 10) hits home, having worked on fusion since my senior thesis at Forrestal long ago. Technical and scientific challenges are indeed a factor in the long time to develop fusion. But another is the need for a policy that develops fusion energy as an integrated system and fosters competition among fusion approaches.
Realizing a fusion power plant requires connecting the pure science, applied science, and engineering together as an integrated system. Understanding fusion plasmas, as done at PPPL, is crucial. But as pointed out, the current policy virtually ignores the other needed technologies. These include neutron-resistant materials and efficiently heating and containing these plasmas. Ultimately it is these technologies that will determine if fusion leads to an attractive power plant, and they need to be developed concurrently.
Stifling competition hampers innovation, slows progress, and increases risk. If fusion has such potential, why pursue just one approach?
One alternative is “inertial confinement,” in which lasers are used to initiate the fusion reactions. The Department of Energy vigorously pursues this to study the physics of nuclear weapons. Energy development is carried out only because of congressional direction. The Naval Research Laboratory (NRL) is leading that program. The key science and technologies are developed in concert by a team from 25 U.S. institutions. Of course, PPPL scientists and engineers are part of that team. Enough progress has been made that it may be possible to demonstrate net fusion power much sooner than originally thought. But an investment in this approach requires a change in policy.
Many talented scientists are dedicated to harnessing fusion energy. We can make it happen. But we need a policy that enables us to reach that goal. I am cautiously optimistic that the new administration may be headed in that direction.
Stuart G. Hibben ’48
8 Years AgoThe news that the Princeton Plasma Physics Lab (PPPL) is back in full action again (cover story, June 10) is the best from Princeton in recent memory. Despite the lost “mothball” years, I am confident that it will again play a lead role in the development of fusion power.
The potential benefits of fusion power are incalculable. To cite just one, it may become the solution to the problem of world population, which is now growing at an unsustainable rate. This linkage was suggested a generation ago by the late futurist Herman Kahn at the Hudson Institute, who surmised that cheap commercial fusion power would become a practical reality in this century, available worldwide.
This should lead to a general rise in the standard of living, notably in third-world countries. Kahn observed that historically, higher standards of living have resulted in lower birth rates. Barring natural or man-made disaster, Kahn’s model thus shows world population peaking and then leveling off after fusion is in place. What a wonderful legacy that would be for the fusion pioneers!
We should all be pleased and proud that PPPL is once again fully engaged in this vital effort.