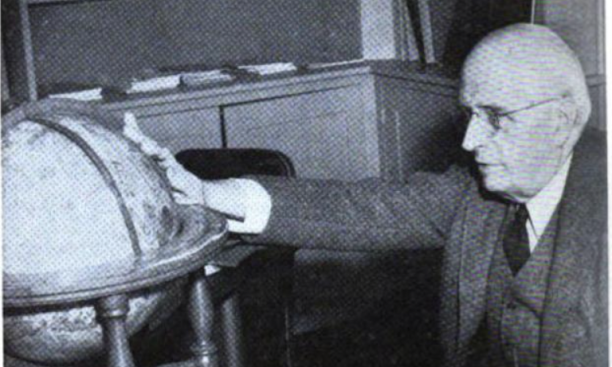
“The force from which the sun draws its power has been loosened against our enemy who has brought war to the Far East.”
With these words, on that fateful day in August, 1945, President Truman announced that an atomic bomb had been dropped over Hiroshima. At the same time he pointed out the inter-relation between astronomy and nuclear physics. The first understanding of a specific means by which atomic power can be released was obtained from the study of the sun’s energy and how it is produced.
The close association between astronomy and basic physics, dramatized in this one instance, has been going on for thousands of years. It was in the sky that man first found the orderliness of nature that his mind now extends to all phenomena. Newton’s law of gravitation, one of the first of the all-embracing natural laws found by the physicists, was based on astronomical observations, and was originally verified by such observations. Astronomers, not physicists, made the measurements which have finally overthrown Newton’s theory in favor of Einstein’s . Thus astronomy has rightfully been called the “Queen of the Sciences.”
It is therefore natural that Princeton, which emphasizes mathematics and physics, should also give strong support to astronomy. In the past the Astronomy Department has had an outstanding reputation, and it aspires to continue this high tradition. Like most departments, this one has two major objectives: carrying out an active instructional program on both the undergraduate and graduate levels, and contributing to man’s scientific knowledge by vigorous and significant research.
The undergraduate instruction is designed to familiarize students with the general scope of astronomical knowledge, especially with the vastness of the physical universe which astronomers have revealed. In addition, astronomy shows in the clearest way how the complicated physical world is subject to fixed and simple natural laws. Thus astronomy is distinctly a cultural course, which helps students to obtain the proper perspective on the earth and its problems. The major course offered by the department is taught by John Q. Stewart ’15 and is taken each year by about 75 upperclassmen. The number of departmental students in astronomy each year rarely exceeds one or two, and the department can offer these a somewhat individualized program, adapted to the needs of each man. The program of graduate instruction has a simpler objective – to train first-rate astronomers. The market for astronomers is not extensive, and only one or two new graduate students are admitted each year. However, some of the country’s leading astronomers have been trained here, including Harlow Shapley and Donald Menzel, director and associate directors, respectively, of the Harvard College Observatory. The department attaches great emphasis to its graduate program, and hopes to maintain this at an active level.
A department which specialized in teaching alone would soon grow stagnant, and in astronomy research has had strong emphasis for many years. A large number of fascinating and significant questions have been studied – and partly answered – by Princeton astronomers. A glimpse at one major problem treated by each of the present members of the department will indicate the type of research activity that constitutes Astronomy at Princeton.
What is the sun made of?
Henry Norris Russell, who retired last year as chairman of the department, is one of the most distinguished scientists in this country, and perhaps the best known astronomer in the world today. In the course of his 41 productive years on the Princeton faculty he has published more than 150 papers on an immense variety of subjects, ranging from “A Planetary Date for Chaucer’s Troilus,” to “The Present State of the Theory of Stellar Evolution.” One of his most celebrated works of research concerned the chemical composition of the sun. When light from a gas is split up into its colors by a spectroscope, different elements in the gas are revealed by the different colors they have sent out. Thus the study of the light from the sun makes it possible to investigate what elements are present in the sun’s atmosphere. This study had been carried on in a general way by Charles A. Young, Professor Russell’s predecessor, who came here from Dartmouth in 1877, lured to Princeton partly by the acquisition of the 23-inch telescope, still our major observational tool, and partly by the construction of the present Observatory office and residence on Prospect Street.
Professor Russell’s contribution was to bring exact physical theory to this investigation. His analysis of the basic laws governing atomic structure led to a mathematical determination of the amount of light in each particular color which the atoms of an element send out; his work along these lines forms one of the classic subjects of pure physics. The application of this analysis to the sun made it possible for the first time to specify the relative amounts of the different chemical elements present. His detailed recipe for the solar atmosphere is known to astronomers as “Russell mixture” and has been widely used in theoretical work on the sun and stars. AN abridged form of this recipe, with all the different metals grouped together, is as follows:
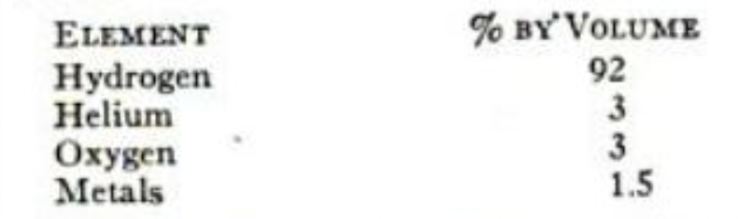
The surprisingly overwhelming abundance of hydrogen which he found has since been confirmed by others for stars in general, as well as for the sun, and has had a profound influence on all of astronomy.
Further work on this significant problem has been carried out by Martin Schwarzschild, who came to Princeton last year from Columbia. Among his research projects was the determination of the chemical composition of the interior of the sun. Since the center of the sun is at a temperature of about 45,000,000°F, and at an almost inconceivable pressure, a cynic might remark that no one is going to get a direct check on Mr. Schwarzschild’s work for some time. But while we cannot go down inside the sun, we have available mathematical and physical laws, and these can provide an “analytical boring machine” for probing deep into the sun. The laws the govern the release of atomic energy in the sun, and the general equilibrium of matter in the sun, are known, and form these it is possible to deduce theoretically what the composition must be to give the sun its observed radius and brightness. The result of the rather involved calculations is simple. The interior of the sun must consist of large quantities of hydrogen and helium, in addition to the heavier elements represented in the Russell mixture. This confirms, for the solar interior, Professor Russell’s discovery of the great abundance of hydrogen and helium in the solar atmosphere.
How do stars evolve?
The birth, growth, decay and death of a star is a subject which has been considered by many astronomers. For many years Professor Russell has been the leading authority on this subject. More recently, considerable interest has been attached to a new phase of this question – can stars be forming at the present time? This problem has been under study by the author, who came from Yale to Princeton a year ago to succeed Professor Russell as chairman of the department. There is strong reason to believe that some very brilliant stars – called supergiant stars – are very young; the reason is simply that they are so bright that they could not have been burning very long without using up all their fuel. These stars are found only in those regions where clouds of matter (see cut) are drifting about between the stars. Hence one naturally suspects that supergiant stars are somehow created from the clouds.
The author has investigated how the observed interstellar clouds might condense into stars. Such clouds are enormously rarefied; if the amount of air breathed in by a fly were expanded to fill a vacuum chamber as big as the Empire State Building, the density resulting would still be many thousands of times greater than that in a typical interstellar cloud! Thus the physical condition of these clouds is quite unusual. A preliminary analysis seems to indicate that known physical forces can lead to the condensation of stars out of interstellar clouds within a reasonable time – a few million years.
How big is a star?
An astronomer who wises to measure all the properties of a particular star seems, by comparison with the physicist, hopelessly handicapped. He cannot bring the star into his laboratory, and even with the most powerful telescopes any star looks like a twinkling point of light, rather than a disc. Fortunately, Nature has been kind to the astronomer and provided him with numerous “double stars,” composed of two stars which go around each other, like the earth around the sun, held together by their mutual gravitational attraction. In some cases, the orbit is such that one star gets in front of the other once each revolution, and an eclipse of a star results. Such double stars, called “eclipsing variable stars,” constitute a veritable mine of information for the astronomer. The two stars are so close that they appear as a single star in even the most powerful telescopes, but measurement of the way the light varies gives the relative sizes of the two stars and, together with other data, makes it possible to “weight” each star separately, and thus to state how much matter it contains.
The analysis of eclipsing variables is largely a Princeton product. The theory and method of analysis was worked out by Professor Russell in 1912, and for many years the most precise measurements on the variation of this light were those made by his colleague, the painstaking, accurate Raymond S. Dugan. The Princeton telescope has for many years been used exclusively for this work.
The recent development of photo-electric cells and electronic techniques permits much more accurate measurement of the light variation in an eclipsing system than has hitherto been possible. Newton L. Pierce, who studied under Professor Dugan, and who is carrying on the Princeton tradition is now designing equipment to achieve the highest possible accuracy in this type of measurement. Since the transmission of light through the earth’s atmosphere fluctuates in an irregular way, he plans to use two photoelectric cells, one for measure the changing light from an eclipsing variable, the other for measuring the light from a constant star nearby. Atmospheric changes should affect both of these measurements in the same way, and thus a comparison of the two sets of measurements should indicate exactly how much the light from the eclipsing variable is actually changing. If an accuracy of a tenth of one per cent can be obtained by this method, completely new vistas of astronomical research may be opened.
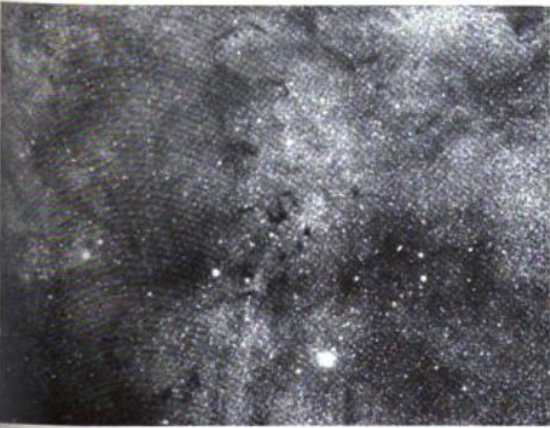
Can science explain society?
There has been increasing discussion of the great contribution which the methods of the physical sciences might make in other fields, especially in the science of society. Active research in this direction has been carried out by Mr. Stewart, who has been a member of this department since 1921. While research of this type necessarily cuts across divisional lines, sociology resembles astronomy in that the astronomer, unlike the physicist or chemist, cannot control the phenomenon which he observes.
One of the most striking results obtained by Mr. Stewart is that the influence of one population on another can be treated, in a wide variety of applications, as similar to the mutual gravitational energy of two planets. The formula is P times p divided by d, where P and p are the two numbers of people and d is the distance in miles between them. Professor Stewart calls this quantity Pp/d the “demographic energy.” For example, the number of students in Princeton from each state is proportional to the product of the state’s white male population P and the total number p students at Princeton, divided by the distance d of each state. Similar results have been obtained for students at other privately endowed colleges and schools, for visitors to a fair, for newspaper subscribers, and even for obituaries from particular cities in the New York Times. Also, demographic energy seems to give a measure of economic wealth. From 1829 and 1939 the demographic energy of all the inhabitants of the United States, computed from census counts, was closely proportional to the national income, both increasing by a factor of about 75 during this period.
These results have naturally aroused considerable interest among social scientists. The School of Economics of the Institute for Advanced Study has been lending a statistical assistant to work at the Observatory with Mr. Stewart. While it is yet too early to assess the ultimate significance of this highly original and path-breaking research, it is possible that further study along these lines may open completely new fields for profitable investigation, and may contribute substantially to man’s understanding of man.
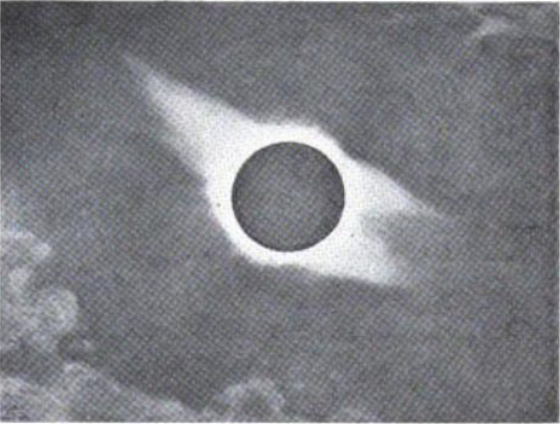
Plans for the Future
The active research program now underway will, of course, be continued. In addition, it is hoped that Princeton’s role as a center of theoretical astrophysics may be expanded. For several decades this country has been pre-eminent in observational astronomy, thanks to its well-equipped observatories, but in the field of theory we have relied heavily on European astronomers. It is believed that the development of an active center of theoretical research at Princeton will not only make our graduate instruction vastly more effective but will help to encourage a more balanced program of astronomical research in the United States. The development of such a center would involve bringing other astronomers here as visiting professors, lecturers, and fellows. A frequent and detailed exchange of outlook between astronomers with widely different experience should bring theory and observation together and help to guide research in the most profitable directions. Since Princeton is already internationally famous for Professor Russell’s work in theoretical astrophysics, such a development would form a logical extension of the Princeton tradition.
Specific steps are underway for the development of a program along these lines. At the present time John E. Merrill of Hunter College, New York, is spending the year in Princeton as research associate in astronomy; he is completing a set of tables that will be widely used in the analysis of eclipsing variable stars. Next year two visiting fellows and about four graduate students are expected at the Observatory, in addition to a visiting professor in the spring term. Some modification of the present offices will be required to accommodate this increased number, and in time a new building will probably be needed. In planning this expanded program it is expected that the experience and interest of the newly appointed Advisory Council will prove very helpful. This Council includes Arthur W. Butler ’92, Bernard Peyton ’17, and David McW. Ludlum ’33. Within the years ahead of Princeton has a great opportunity to make a unique and significant contribution to the development of astronomy.
The telescope at the New Observatory, the major piece of equipment used for astronomical research at Princeton, is the subject of this week’s cover photograph taken by Alan W. Richards. In operation, light from a distant star passes through the lens, 23 inches in diameter at the end of the 30-foot tube, and comes to a focus at the eyepiece. The floor of the Observatory can be raised or lowered by a button control for the convenience of the observer. New photoelectric equipment, designed to measure precisely the weakest light waves, is now being obtained.
Most of the astronomical research at Princeton, however, is theoretical, and covers such a variety of subjects as the birth of stars and the chemical composition of the sun’s interior. This work, together with the general objectives and plans of the Department of Astronomy, is described in the accompanying article by Lyman Spitzer Jr., departmental chairman. Like many other U.S. astronomers, Mr. Spitzer was trained originally at Princeton, receiving his Ph.D. in 1938.
This was originally published in the March 19, 1948 issue of PAW.