

Most organisms produce offspring to pass on their genetic information and continue the species. Some bacteria and plants achieve this by budding or cloning, creating exact copies of themselves. More advanced plants and animals reproduce sexually, with two organisms combining their DNA — half from one parent, half from the other — into a completely new genetic being. Then there’s Oxytricha, a pond-dwelling organism with a complicated sex life.
Oxytricha is able to reproduce asexually to create a nearly exact clone of itself. But it also has sex — not to produce offspring, but to mix its DNA with a partner and change both organisms in the process. “Afterward, the organisms may look physically the same, but are completely rejuvenated with a brand-new genome,” says Professor Laura Landweber ’89.
Landweber has spent the past decade exploring just how Oxytricha achieves this amazing feat, a process that could lead to better understanding how our own genome replicates and breakthroughs in treatment of diseases including cancer. An undergrad at Princeton in molecular biology, Landweber returned in 1994 as an assistant professor and is now the only female full professor in the ecology and evolutionary biology department. She’s been interested in the surprisingly rich life of single-celled organisms ever since looking into a microscope in her parents’ basement. “These pond-dwelling organisms are seemingly simple, but when you look inside their genomes they are as complex as us, if not more so,” she says.
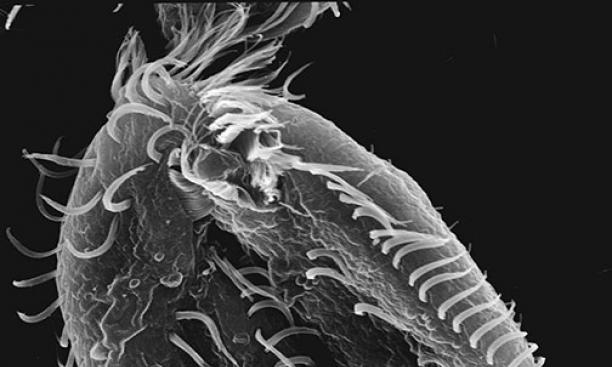
Case in point: Oxytricha, a paramecium-like oval covered in tiny hairs that has two separate nuclei, each with its own DNA. The larger nucleus, called the macronucleus, controls day-to-day activity within a cell and has more than 16,000 chromosomes. Each one is present in 2,000 copies, for a total of some 32 million. The smaller nucleus, or micronucleus, is used only during sexual conjugation and is even more of a mess, containing a quarter of a million individual gene pieces, spliced and encrypted in a chaotic pattern. “It’s like taking long sentences and breaking them into words, and then hiding those words in different places until you create gibberish,” Landweber says.
The magic happens when two Oxytricha combine during sex. Each breaks open this encrypted archive of the micronucleus and somehow assembles it back into a completely new set of chromosomes for the macronucleus, jettisoning 90 percent of the DNA as so much junk. Landweber compares it to “having a really good editor who restores everything to its original state every time.”
The keys to this editorial tour de force, Landweber’s lab has discovered, are millions of molecules of RNA, which ordinarily help convert the information in DNA into proteins. In this case, however, the RNA marks the genes so that they can be reassembled perfectly. Landweber hypothesizes that Oxytricha developed this strange reproductive ritual as a response to virus-like invaders called transposons that wreak havoc with the DNA of microorganisms. By sequestering the “working” set of chromosomes in the macronucleus, the organism can protect them from harm. Meanwhile, the organism allows the transposons to roam free in the micronucleus, knowing the RNA proofreaders can always put scrambled DNA back together.
Oxytricha isn’t the only organism containing this type of RNA, known as non-coding RNA. Humans and other organisms also contain these molecules, which geneticists speculate may play a role in keeping DNA stable and its code properly arranged. If that’s true, a malfunction of these molecules could play a role in diseases such as cancer, where the genome is rearranged or unstable. “Understanding how this RNA works can help us understand the rules by which genomes either rearrange or remain stable over a lifetime,” says Landweber. In other words, studying this complex pond-dweller may not give humans the key to rejuvenating their genomes, but it may allow us to prolong lives in cases where genomes would cut them short.