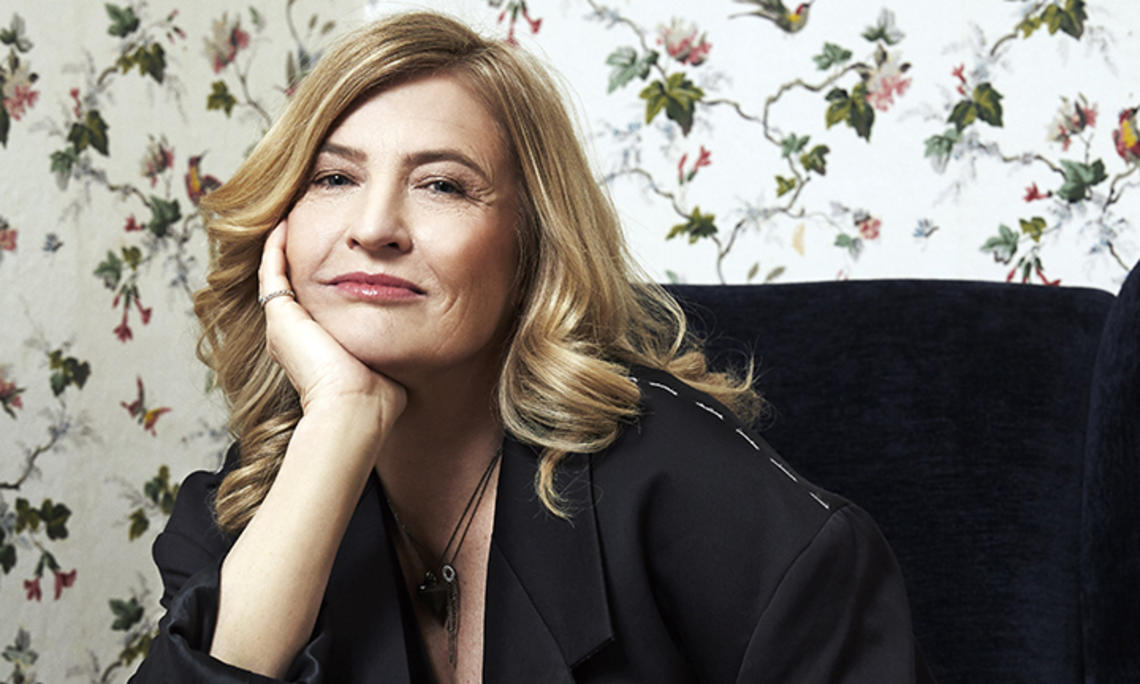
Katherine Freese ’77, a physicist at the University of Texas, Austin, has proposed that dark matter wasn’t created in the Big Bang along with all other matter, but rather in a second Big Bang some weeks later. Freese, who was recently elected to the National Academy of Sciences and serves as director of the Weinberg Institute for Theoretical Physics, spoke with PAW about the Dark Big Bang theory and what it means for our understanding of the universe.
What is dark matter?
All the stuff of our daily experience, our bodies, the air we breathe, the walls, the stars, and planets, everything that we’re familiar with — all of that is made of atoms. But if you add all of that together, it is only about 5% of the content of the universe. The other 95% (the part you don’t see) consists of 25% dark matter and 70% dark energy. The dark side is the dominant content of the universe. So you can bet we’re trying to figure out exactly what it is.
Our galaxy, for example, is almost entirely made of dark matter. It’s like 95% dark matter, and the ordinary stuff that makes up the stars is the remainder. The stellar material is all in a flat surface that we call a disc. Imagine a Frisbee inside a giant basketball and the Frisbee is where the ordinary stars are; they’re actually along spiral arms like a pinwheel and the sun is along one of these arms. So that is where almost all the stars are, on this flattened disc, then there’s this giant sphere around the whole thing, and that is where it’s almost all entirely made of dark matter.
Where does the second Dark Big Bang Theory fit?
According to the standard paradigm the dark matter particles are produced in the Big Bang alongside ordinary matter and light. This assumption roots in the belief that the dark and the visible world are closely connected. The known elementary particles which make up the visible matter interact via one or several of the following fundamental forces: the strong force, the electromagnetic force, and the weak force.
Gravity is so much weaker than these three forces that it plays no role in the microscopic world of elementary particles. While the inert nature of dark matter rules out that it feels the strong or the electromagnetic forces, it has long been thought that dark matter interacts with ordinary matter via the weak force. Intriguingly, the neutrino — a particle we know from radioactive decays — has precisely the property of interacting solely through the weak force. Unfortunately, neutrinos are so light that they cannot easily be bound in astrophysical structures. Neutrino dark matter would jeopardize the formation of galaxies in the early universe. A hypothetical heavier cousin of the neutrino — dubbed the Weakly Interacting Massive Particle (WIMP) — would support galaxy formation and is often considered as the leading dark matter candidate.
The prospect of detecting WIMP dark matter has stimulated unprecedented experimental efforts over the last decades. In fact, my work in the 1980s together with David Spergel, the former Chair of Princeton’s Astronomy Department, pioneered the calculations that led to these experiments. Thanks to the predictions of our work, detectors have now been built worldwide — in the US, Canada, Europe, Korea, China, and even at the South Pole. In dedicated underground laboratories, shielded against cosmic radiation by kilometers of rock, an army of physicists have searched for the recoil produced by dark matter scattering off atomic nuclei. If dark matter is subject to the weak force, the rate of such recoils can be predicted and compared against experimental data. Unfortunately, not a single dark matter scattering event has ever been confirmed (one Italian experiment named DAMA has claimed detection using a technique proposed by Drukier, Spergel, and me, but must be checked by other similar detectors). The searches, nevertheless, continue with ever greater precision — an important effort since there exist variations of WIMP dark matter which give rise to a suppressed recoil signal. A discovery of WIMP or WIMP-like dark matter could, therefore, still be just around the corner.
The Big Bang has long been considered as the origin not only of the visible, but also of the dark matter. However, this standard paradigm breaks, if the two forms of matter only interact gravitationally. Gravity is too weak to transform matter and light into dark matter — even in the hot dense state of the early universe. If dark and visible matter are fundamentally distinct, if they do not share any of the forces shaping the microscopic world, they are subject to their own separate histories.
But what could have produced the dark matter if the not the Big Bang? The most plausible possibility is that there has been a second Big Bang, which we call the Dark Big Bang, that took place not long after the Big Bang. Just as the Big Bang converted the energy of empty space — the vacuum energy — into matter and radiation, the Dark Big Bang converted a second type of vacuum energy (dark vacuum) into dark matter. An exciting new idea to search for dark matter is to search for the Dark Big Bang. (To read more: https://iai.tv/articles/katherine-freese-martin-winkler-there-were-two-big-bangs-auid-2545)
Why search for dark matter? Why is this important?
One is, the reason the human species survives is because we ask questions. What is out there? What is around us? We’re a curious species. And that has to do with who we are. And it’s one of the big questions of humanity, in my opinion, discovering what the majority of the Universe is made of. These are big questions that we have to answer, because that’s who we are. And this question, the answer is the curiosity of the species.
Also, this has led to the survival of the species, because every time there’s something new that is out there, we end up figuring out a way to make it helpful to humanity. — Interview conducted and condensed by Nicholas DeVito
0 Responses